Hendrik Huwald
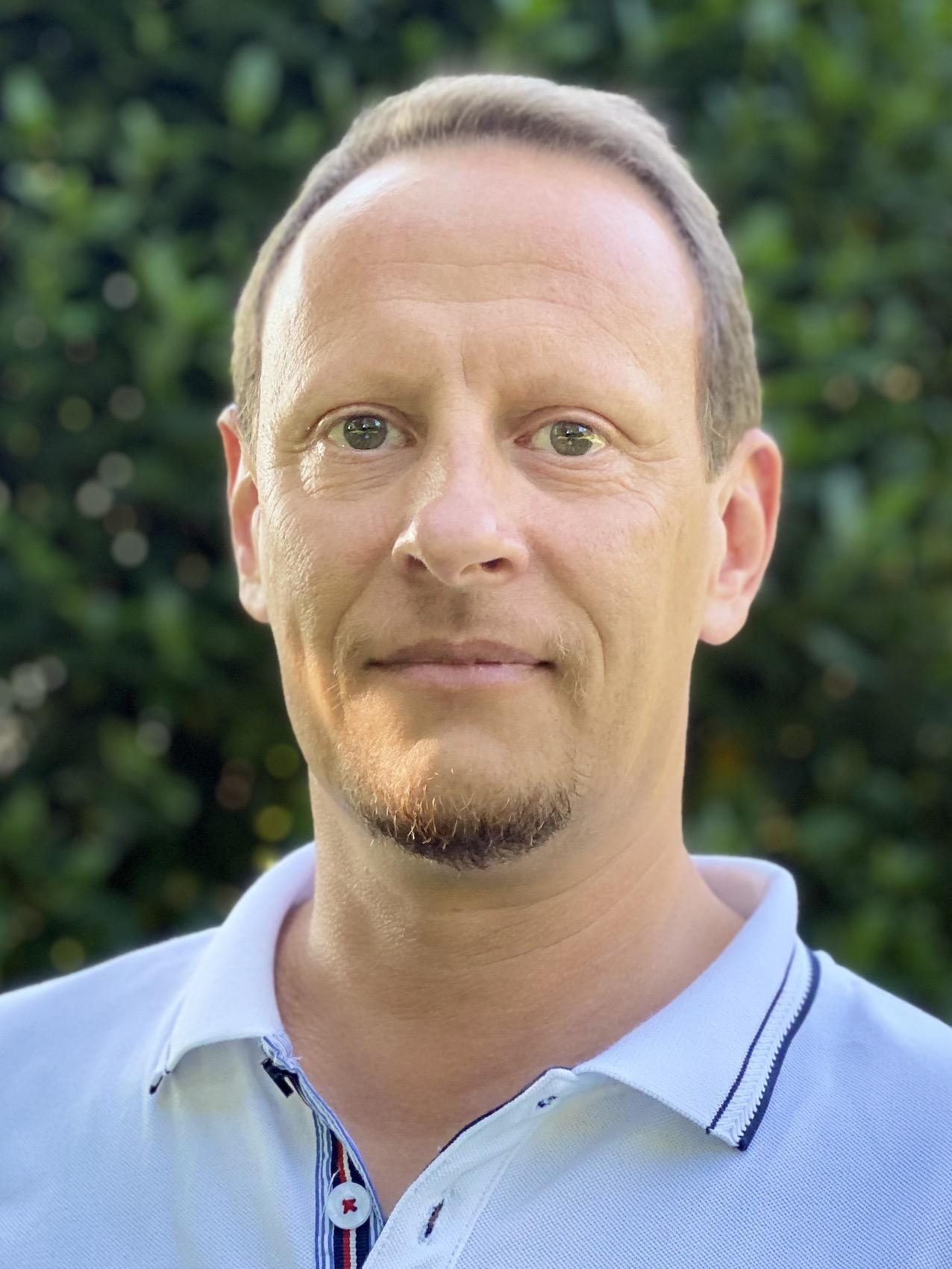
EPFL Valais Wallis
EPFL ENAC IIE CRYOS
Route des Ronquos 86
1951 Sion
Web site: Web site: https://cryos.epfl.ch
EPFL ENAC SSIE-GE
GR A0 444 (Bâtiment GR)
Station 2
1015 Lausanne
Current work
- Surface mass and energy balance in alpine & polar environments
- Stream temperature dynamics and climate change
- Measurement techniques and sensor development
- Environmental monitoring and analysis
Biography
Dr. Hendrik Huwald is a scientist at the Laboratory of Cryospheric Sciences (CRYOS) and a lecturer at the Environmental Sciences and Engineering Section (SSIE) of the Swiss Federal Institute of Technology in Lausanne (EPFL). He completed his PhD in 2003 at the Swiss Federal Institute of Technology in Zürich (ETHZ), developing a numerical model for sea ice and studying energy transfer processes in the Arctic. Between 2005 and 2012 he was a post-doctoral fellow and scientist at the Laboratory of Environmental Fluid Mechanics and Hydrology (EFLUM) of EPFL working on Alpine snow-atmosphere interaction, and energy balance-related research mainly in mountain regions. During this time, he designed, led and participated in numerous large field experiments in different environments. In 2013 he joined the newly founded CRYOS laboratory where he conducted research in the domains of snow science, hydrology, boundary layer meteorology and environmental sensing. He has extensive experience with project management both on national and international level.Education
PhD
Climatology
Inst. for Atmospheric and Climate Sciences, ETH Zurich
2003
MSc
Climatology
Inst. for Atmospheric and Climate Sciences, ETH Zurich
1999
BSc
Geography, Meteorology
J.W.Goethe University, Frankfurt
1995
Publications
Infoscience publications
Publications
[36] Influence of air flow features on alpine wind energy potential
Frontiers In Energy Research. 2024. DOI : 10.3389/fenrg.2024.1379863.[35] Using the Sensible Heat Flux Eddy Covariance-Based Exchange Coefficient to Calculate Latent Heat Flux from Moisture Mean Gradients Over Snow
Boundary-Layer Meteorology. 2024. DOI : 10.1007/s10546-024-00864-y.[34] Quantifying urban climate response to large-scale forcing modified by local boundary layer effects
Frontiers in Environmental Science. 2024. DOI : 10.3389/fenvs.2024.1438917.[33] Evidence of Strong Flux Underestimation by Bulk Parametrizations During Drifting and Blowing Snow (vol 182, pg 119, 2022)
Boundary-Layer Meteorology. 2023. DOI : 10.1007/s10546-023-00809-x.[32] Combining Weather Station Data and Short-Term LiDAR Deployment to Estimate Wind Energy Potential with Machine Learning: A Case Study from the Swiss Alps
Boundary Layer Meteorology. 2023. DOI : 10.1007/s10546-023-00808-y.[31] Correction to: Evidence of Strong Flux Underestimation by Bulk Parametrizations During Drifting and Blowing Snow
Boundary-Layer Meteorology. 2023. DOI : 10.1007/s10546-023-00809-x.[30] Observations and simulations of new snow density in the drifting snow-dominated environment of Antarctica
Journal Of Glaciology. 2022. DOI : 10.1017/jog.2022.102.[29] Future water temperature of rivers in Switzerland under climate change investigated with physics-based models
Hydrology and Earth System Sciences. 2022. DOI : 10.5194/hess-26-1063-2022.[28] Evidence of Strong Flux Underestimation by Bulk Parametrizations During Drifting and Blowing Snow
Boundary-Layer Meteorology. 2022. DOI : 10.1007/s10546-021-00653-x.[27] Climate change scenarios at hourly time‐step over Switzerland from an enhanced temporal downscaling approach
International Journal of Climatology. 2021. DOI : 10.1002/joc.7032.[26] Changement climatique et température des rivières
Aqua & Gas. 2021.[25] Radar measurements of blowing snow off a mountain ridge
The Cryosphere. 2020. DOI : 10.5194/tc-14-1779-2020.[24] Stream temperature and discharge evolution in Switzerland over the last 50 years: annual and seasonal behaviour
Hydrology and Earth System Sciences. 2020. DOI : 10.5194/hess-24-115-2020.[23] The European mountain cryosphere: a review of its current state, trends, and future challenges
The Cryosphere. 2018. DOI : 10.5194/tc-12-759-2018.[22] How do Stability Corrections Perform in the Stable Boundary Layer Over Snow?
Boundary Layer Meteorology. 2017. DOI : 10.1007/s10546-017-0262-1.[21] Influence of slope-scale snowmelt on catchment response simulated with the Alpine3D model
Water Resources Research. 2017. DOI : 10.1002/2017WR021278.[20] StreamFlow 1.0: an extension to the spatially distributed snow model Alpine3D for hydrological modelling and deterministic stream temperature prediction
Geoscientific Model Development. 2016. DOI : 10.5194/gmd-9-4491-2016.[19] Adapting tilt corrections and the governing flow equations for steep, fully three-dimensional, mountainous terrain
Boundary-Layer Meteorology. 2016. DOI : 10.1007/s10546-015-0066-0.[18] Fliessgewässertemperatur - zwei Modellansätze für zukünftige Prognosen
Aqua & Gas. 2016.[17] Attenuation of wind-induced pressure perturbations in alpine snow
Journal of Glaciology. 2016. DOI : 10.1017/jog.2016.53.[16] Comparison of different numerical approaches to the 1D sea-ice thermodynamics problem
Ocean Modelling. 2015. DOI : 10.1016/j.ocemod.2014.12.006.[15] Stream temperature prediction in ungauged basins: review of recent approaches and description of a new physics-derived statistical model
Hydrology and Earth System Sciences. 2015. DOI : 10.5194/hess-19-3727-2015.[14] Flow during the evening transition over steep Alpine slopes
Quarterly Journal of the Royal Meteorological Society. 2013. DOI : 10.1002/qj.1985.[13] Thermal diffusivity of seasonal snow determined from temperature profiles
Advances in Water Resources. 2013. DOI : 10.1016/j.advwatres.2012.06.011.[12] Carbon monoxide as a tracer of gas transport in snow and other natural porous media
Geophysical Research Letters. 2012. DOI : 10.1029/2011GL050247.[11] Fiber optic distributed temperature sensing for the determination of the nocturnal atmospheric boundary layer height
Atmospheric Measurement Techniques. 2011. DOI : 10.5194/amt-4-143-2011.[10] Evolution of superficial lake water temperature profile under diurnal radiative forcing
Water Resources Research. 2011. DOI : 10.1029/2011WR010529.[9] Field study of the dynamics and modelling of subgrid-scale turbulence in a stable atmospheric surface layer over a glacier
Journal of Fluid Mechanics. 2010. DOI : 10.1017/S0022112010004015.[8] Stream Temperature Response to Three Riparian Vegetation Scenarios by Use of a Distributed Temperature Validated Model
Environmental Science & Technology. 2010. DOI : 10.1021/es902654f.[7] Albedo effect on radiative errors in air temperature measurements
Water Resources Research. 2009. DOI : 10.1029/2008WR007600.[6] Estimation of wet surface evaporation from sensible heat flux measurements
Water Resources Research. 2009. DOI : 10.1029/2008WR007544.[5] Subgrid-Scale Dynamics of Water Vapour, Heat, and Momentum over a Lake
Boundary-Layer Meteorology. 2008. DOI : 10.1007/s10546-008-9287-9.[4] Spatial pattern and stability of the cold surface layer of Storglaciaren, Sweden
Journal of Glaciology. 2007. DOI : 10.3189/172756507781833974.[3] Distributed fiber-optic temperature sensing for hydrologic systems
Water Resources Research. 2006. DOI : 10.1029/2006WR005326.[2] A multilayer sigma-coordinate thermodynamic sea ice model: Validation against Surface Heat Budget of the Arctic Ocean (SHEBA)/Sea Ice Model Intercomparison Project Part 2 (SIMIP2) data
Journal of Geophysical Research. 2005. DOI : 10.1029/2004JC002328.[1] Reconciling different observational data sets from Surface Heat Budget of the Arctic Ocean (SHEBA) for model validation purposes
Journal of Geophysical Research. 2005. DOI : 10.1029/2003JC002221.Teaching & PhD
Teaching
Environmental Sciences and Engineering